Introduction
During the formation process of lithium battery:
- The SEI film on the graphite surface of the negative electrode is mainly composed of inorganic lithium salts {Li 2 O, LiX (X=F, Cl, etc.)} in the early stage of formation.
- The layer structure is relatively dense. And it is more stable to electrolyte and high temperature performance, while the outer layer of SEI film is mainly composed of organic lithium salts (ROCO 2 Li, ROLi, etc.), the structure is loose, and the performance is not stable enough.
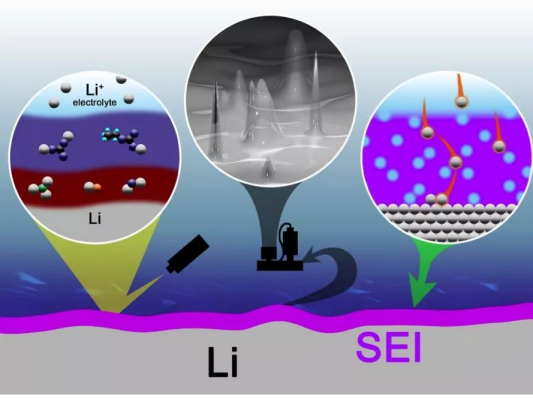
1. Analyze of the Influence of Chemical Charge Cut-off Voltage on Battery Performance
1.1 Influence of formation cut-off voltage of lithium cobalt oxide battery on battery performance
For lithium cobalt oxide (LiCoO 2) as the positive active material and graphite as the negative active material to form a battery system, by adjusting the formation charge cut-off voltage, the influence of the formation process on the battery performance was investigated. The comparative experiment process is as follows:
1. Through slurry mixing, the 683064 soft pack lithium-ion battery is assembled through the steps of coating, drying, assembling, liquid injection, standing, forming, and dividing.
2. The battery is divided into two groups, A/B. The cut-off voltage of group A is 3.8V, and the cut-off voltage of group B is 3.7V.
3. Comparative analysis of battery capacity, initial efficiency, energy density, internal resistance, rate, impedance, high temperature storage and cycle performance.
Buy Now: SEMCO Battery Charge & Discharge Cabinet Tester SI BCDS 99V – 20A – 1CH.
Basic Performance Comparison
The performance comparison results are shown in the following table. The capacity, specific capacity, initial efficiency, energy density, and battery gas production deformation rate of the battery formed by the low cut-off voltage are better than those of the high cut-off voltage, because the positive electrode specific capacity of the two schemes A/B is the same. the difference of the whole battery mainly comes from the change of the negative electrode. The active lithium ion consumed by the B scheme is less than that of the A scheme, which makes the first efficiency and capacity higher, and the overall thickness is smaller.
mAh | mAH.g-1 | % | mm | Wh. L-1 | |
A | 1947.6 | 160.7 | 89.6% | 5.29 | 574.9 |
B | 1947.9 | 162.1 | 90.8% | 5.21 | 509.2 |
Comparison of battery performance with two chemical cut-off voltages.
EIS Comparison
The difference in the internal resistance properties of the cells mainly comes from the membrane impedance and ion transfer impedance. Because the thickness of the SEI film under the B process condition is relatively thin, the membrane impedance and ion transfer impedance are both lower than those of the A process condition. The specific test results are shown in the figure below. :

EIS Comparison of Two Processes
1.2 Influence of Formation Depth of Lithium Iron Phosphate Battery on Battery Performance
Take olivine-type LiFePO 4 as the positive electrode material, graphite as the negative electrode material, ternary electrolyte ethylene carbonate (EC), diethyl carbonate (DEC), and methyl ethyl carbonate (EMC) in a volume ratio of 1:1: A 1865140 square power battery with a rated capacity of 10Ah was assembled with LiPF 6 with a supporting electrolyte of 1 mol/L in a ratio of 1 . The formation depth is controlled by adjusting the formation time, and the electrical properties of the battery are tracked and tested, and the influence of different formation depths on the electrical performance of the battery is analyzed.
By adjusting the formation charging time, the formation depths of the batteries of Groups I to IV are controlled to be 40%, 60%, 80% and 100% of the rated capacity, respectively.

Influence of battery internal resistance
The change of the internal resistance of the battery is shown in the figure below. With the deepening of the formation depth, the internal resistance of each battery group shows a decreasing trend after the formation of the battery. The electrode activation of the battery with a shallow formation depth is incomplete, and the reaction between the electrolyte and the electrode material is not sufficient. , a complete and dense SEI film has not been formed on the electrode surface, and

SEI film has a large impedance.
Changes in the average internal resistance of each group of batteries.
The effect of high temperature cycle life of batteries.
The cycle life curve of each group of batteries at 1 C in a constant temperature environment of 45 °C is shown in the figure below. The cycle life of batteries in groups II to IV is basically the same as the change rate of the number of charges and discharges. After 100 cycles, the capacity of the batteries in group IV remained unchanged. The capacity of the battery of group I is relatively fast, especially after the 30th cycle, the capacity of the battery of group I has dropped significantly, and the capacity retention rate after 100 cycles is only 97.51%. It shows that when the formation depth reaches more than 60% of the rated capacity, continuing to deepen the formation depth has little effect on the high temperature cycle performance of the battery.
2. The Effect of Pressure on the Formation Process
The pressurization mentioned here refers to soft-packed cells, and other types of cells are not discussed. Since the soft-packed battery adopts an aluminium-plastic packaging structure, its external structure determines that the pole pieces cannot be closely arranged, and the pole pieces are easy to be arranged. There are voids, and the gas generated during the battery formation process is also easy to remain between the pole pieces. In the subsequent Degas sealing, the gas cannot be completely discharged, thus affecting the battery performance. Therefore, it is considered to adopt the rolling process between the two charges. Remove the gas between the pole pieces.
The comparison system is a lithium iron phosphate soft-packed cell, the separator of the battery is a 25 μm polypropylene porous single-layer separator, and the electrolyte is 1 mol/L LiPF 6 /(EC+EMC+DMC) (volume ratio 1:1:1), divided into There are two groups of A/B, in which the cells of group A are not pressurized and exhausted during the formation process, and the cells of group B are added to the process of pressurization and exhaust during the two charging processes of formation, and the pressure of group B is B1 ( The pressure value is 4~6N), B2 (the pressure value is 20~30N), and B3 (the pressure value is 50~60N), and the performance of the cells after the formation of the two groups of A/B is compared.

The capacity of the cells after formation is compared, and the comparison results are shown in the following table. The capacity of group A (without pressurization process) is smaller than that of group B (with pressurization process), and the capacity of group B cells increases with the increase of pressure. Increase.

Capacity comparison results of pressurized and unpressurized cells.
The main reason for this is that a large amount of gas will be generated during the formation of lithium-ion batteries, and due to the structure of the pouch cell itself, the gas generated during the formation process will increase the distance between the positive electrode, the separator, and the negative electrode, hindering lithium The transmission of ions from the positive electrode sheet to the negative electrode sheet, in addition, the presence of gas will also hinder the contact of the electrolyte with the positive electrode and the negative electrode, which makes the local wetting performance of the negative electrode worse, and finally leads to a large number of unreacted dead zones on the negative electrode sheet. The presence of dead will increase the possibility of lithium deposition in the battery, and will seriously affect the performance of the battery itself.

Dismantling diagram of the battery cell when the formation process is not pressurized or the pressure is less than the process requirements
3. The Effect of Temperature on the Formation Results of Lithium-ion Batteries
In the process of formation, applying high temperature can reduce the viscosity of the electrolyte, accelerate the diffusion of ions, and ensure that electrons and ions are rapidly combined under high current; formation at high temperature can not only make the SEI film on the surface of the electrode react more fully, but also It can enhance the liquid absorption of the separator, which is beneficial to reduce the inflation of the battery.
However, high temperature formation will reduce the stability of the SEI film, resulting in poor battery cycle performance, because high temperature will aggravate the dissolution of the SEI film and the co-insertion of solvent molecules, while the SEI film tends to be stable at low temperatures. Studies have shown that the formation at low temperature is mainly based on solvent reduction, the reduction speed of lithium salts is slow, and the formation speed of SEI film is slow, so the deposition of solvent products is more orderly and denser, which is more conducive to prolonging the service life of batteries.
In this paper, the flexible packaging lithium-ion battery of lithium cobalt oxide graphite system is taken as the research object, and the effect of temperature on the formation effect in the high temperature pressure formation process is studied. The chemical composition process is divided into 4 cases, the temperature is 50℃, 60℃, 80℃ and the temperature change, the temperature change means that the temperature of the two charging processes is different, the first charge stage is 40℃, the second charge The temperature during the charging phase was 70°C.
The performance of the first 350 cycles is similar, and the initial capacity can reach 3000 mA h, but after 400 cycles, the capacity retention rate begins to differ. The retention rates are 90.57%, 90.63%, 93.44%, and 92.02%, respectively, indicating that the cycle performance of the 80°C high-temperature formation battery is better than that of the other solutions. It may be because the 80°C formation has greater activity of the battery and a thicker SEI film, which is beneficial to the cycle stability of the battery.

For the comparison of high-temperature formation or low-temperature formation, there is no definite result to show that it can be said that high-temperature formation is definitely good, or low-temperature formation is certain. Experiments verify that the formation process parameters suitable for the battery itself are selected.
4. Optimization of the Formation Process of NMC811 High–Nickel Lithium–Ion Battery
Chengyu Mao (first author), Rose E. Ruther (corresponding author), David L. Wood III (corresponding author) and others from Oak Ridge National Laboratory in the United States investigated the formation time of high specific energy battery (NCM811/graphite) (10 The relationship between Li-ion battery performance and lithium-ion battery performance was studied, and it was found that too short formation time would lead to the precipitation of anode metal Li and deterioration of electrochemical performance, while moderate formation time (30h and 26h) would help It is used to reduce the increase in internal resistance of lithium-ion batteries, improve the capacity retention rate, and effectively avoid the precipitation of metal Li.
All batteries in the experiment were first charged to 1.5V (to avoid the corrosion of the negative electrode copper foil), and then left at 30 or 40°C for 6 hours to allow the electrolyte to fully infiltrate, and then formed according to the system shown in the table above (among which, 30h formation is C/2 charge and discharge for the first time, and C/10 charge and discharge for the second time, the label in the table is wrong). The figure below shows the first and subsequent charge-discharge curves of the battery with different formation times. From Figure F below, it can be seen that the first coulombic efficiency of the battery with C/10 rate in the first charge is significantly higher than that with C/2 for the first charge. discharged battery. However, from the perspective of the average Coulomb efficiency, the batteries formed by 30h (2 times of C/2 charge and discharge) and 26h (1 time of C/10 charge and discharge) have the best average Coulomb efficiency, indicating that these two batteries occur in the first charge and discharge. fewer side reactions. However, the average coulombic efficiency of the 86h battery with the longest formation time (4 times of C/10 charge and discharge) is not the highest. This is mainly because at this smaller rate, the negative electrode has a relatively long time at low potential, causing More side reactions and SEI growth, thus reducing the overall coulombic efficiency of the cell.

After the formation, Chengyu Mao immediately dissected the battery and analyzed the changes of the negative electrode (as shown in the figure below). From the figure below, it can be seen that there is no metal Li precipitation in the negative electrode of the battery formed by C/10 small current (86h and 26h formation). However, in the batteries with larger C/2 ratios (30h, 10h and 10h@40°C), obvious metal Li precipitation can be observed on the surface of the negative electrode, while the batteries with 30h formation are in the second the C/10 cycle is used in the second cycle, so the Li deposition is lighter than the two batteries with 10h cycle, which indicates that part of the metallic Li formed during the first charging process is still active and can participate in the electrochemical reaction. This experiment shows that during the first charging process of chemical formation, since the negative electrode has not formed a stable SEI film, the kinetic conditions are poor, so a small current must be used for the first charging to avoid the precipitation of metal Li on the surface of the negative electrode. At the same time, we also found that although the battery at 10h@40 ℃ was soaked at high temperature, it did not alleviate the problem of Li precipitation in the negative electrode. Therefore, this also shows that soaking at 30℃ for 6h can fully ensure that the electrode is fully wetted. For batteries with many or winding structures, the infiltration time should be appropriately extended to ensure sufficient infiltration of the electrodes and separators by the electrolyte.

The following figure shows the electrical performance test results of the batteries after different chemical formation systems. It can be seen from figure a below that although the negative electrode of the battery charged and discharged at a high rate during the formation process has obvious Li precipitation phenomenon, but in the rate test several The performance of these batteries is highly similar, with a capacity of 194mAh/g at low rates, which indicates that the metal Li precipitated during the first charge and discharge is still active and can still be re-inserted into the positive and negative electrodes in subsequent cycles. . Although the rate performance is very close, several batteries have opened a clear gap in cycle performance (Figures B and C below). g, the capacity retention rate is 91.2%. On the contrary, the battery with low current formation (86h formation) has poor cycle performance, and the battery with the shortest formation time (10h formation) has the worst cycle performance, and the capacity retention rate after 300 cycles Only 89.6% (10h) and 88.1% (10h@40℃), the results show that the formation system has a significant impact on the long-term cycle performance of lithium-ion batteries, not that the longer the formation time, the better, the medium time (30h and 26h) ) is more conducive to the improvement of long-term cycle performance of lithium-ion batteries.

Figure C below shows the trend of internal resistance (tested by pulse method) of the 30h and 86h formation batteries during the cycle. It can be seen from the figure that the two batteries have similar internal resistances at the beginning of the cycle, but the formation time is longer in the cycle. The internal resistance of the battery (86h formation) increased significantly faster than that of the 30h formation, resulting in a faster decline in the capacity of the 86h formation, which indicates that the SEI film formed during the long-term formation at a small current cannot effectively passivate the negative electrode surface, which leads to the continuous growth of the negative SEI, causing the loss of active Li and the increase of internal resistance. After the effect of the increase in internal resistance is minimized by low-rate charge and discharge (the results of low-rate charge and discharge are shown in Figure D below), the decrease in battery capacity is mainly due to the loss of active Li and active materials. The batteries of 86h, 30h and 26h the discharge capacity at low current is relatively close, both around 176mAh/g, while the reversible capacity of 10h and 10h@40 ℃ batteries is lower at low current (170 and 166mAh/g, respectively), indicating that the battery formed by high current More active Li and active species will be lost during cycling.

Dissect the battery after the cycle and observe the change of the negative pole piece (as shown in the figure below). It can be seen that no Li precipitation is observed on the surface of the negative electrode of the battery formed at 86h and 26h, indicating that the formation of small current can effectively avoid the formation of metal Li. Precipitation, and 30h chemical formation (C/2 charge and discharge once, C/10 charge and discharge once), there is no obvious Li precipitation phenomenon on the surface of the negative electrode (this is mainly because the second C/10 small current charge and discharge reduces the negative electrode precipitation phenomenon). Li), while the two batteries formed after 10 h had obvious Li precipitation on the surface of the negative electrode.


Conclusion
The SEM test shows that the SEI film formed on the negative electrode surface after 86h formation is thicker than that of the batteries after 30h and 26h formation, which also verifies the previous impedance test results. For the batteries formed at 10h and 10h@40℃, obvious traces of Li precipitation can be observed on the surface of the negative electrode.
More Articles:
Introduction to Cell Balancing System,
Intercontinental Battery Circle,
Application of Isolation testing Technology,
Battery separator material,
History of Lithium Battery Development,
Production problems of lithium batteries,
Aging Mechanisms of Li-ion Batteries.,
Principles of Power Battery System Design,
Battery Pole Piece Coating Process,
Point Defects in Pole Piece Coating,